VIEWS AND PERSPECTIVES

EDITOR'S SUGGESTION
2024, 73 (20): 206201.
doi:10.7498/aps.73.20241129
Abstract +
In recent years, significant progress has been made in the research of plastic thermoelectric materials, for example, Ag2S-based alloys. These materials exhibit excellent room-temperature plasticity due to their low slipping barrier energy and high cleavage energy, with synergistic enhancements in plasticity and thermoelectric properties achievable through alloying and doping strategies. The latest study on Mg3Bi2-based single crystals demonstrated superior performance in terms of plastic deformation capability and room-temperature thermoelectric properties. Microstructural characterization and theoretical calculation have revealed the crucial role of dislocation glide in the plastic deformation process of Mg3Bi2single crystals, especially, the low slipping barrier energy observed in multiple slip systems. Importantly, the Te-doped single-crystalline Mg3Bi2shows a power factor of ~55 μW cm–1K–2andZTof ~0.65 at room temperature along theabplane, which exceed those of the existing ductile thermoelectric materials. These findings not only deepen the understanding of microscopic deformation mechanisms in plastic thermoelectric materials but also establish an important foundation for optimizing material properties and developing novel flexible thermoelectric devices. Future applications of these materials in practical devices still face challenges in thermal stability, chemical stability, and interfacial contact. Addressing these issues will promote the application of plastic thermoelectric materials in the field of flexible electronics.
EDITOR'S SUGGESTION
2024, 73 (20): 207303.
doi:10.7498/aps.73.20241029
Abstract +
Fractional quantum Hall (FQH) states with fractionalized quasiparticles are exotic topologically ordered quantum states driven by strong correlation between particles. Since the first discovery in 1982 in two-dimensional electron gases penetrated by strong magnetic fields, FQH physics has become an attractive frontier of condensed matter physics. Since last year, FQH transport at zero magnetic field has been observed in moiré superlattices based on transition metal dichalcogenides (TMDs) and graphene. Furthermore, the evidence of fractional quantum spin Hall effect has also been reported in TMD moiré superlattices. These results demonstrate that moiré superlattices are an ideal platform for controlling band structures and interactions to realize fractionalized topological states without the intervention of external magnetic fields. In this paper, we will briefly review the recent research progress on fractionalized topological states in moiré superlattices, summarize the existing challenges, and discuss possible future development of this field.
SPECIAL TOPIC—Precision spectroscopy of few-electron atoms and molecules

2024, 73 (20): 202101.
doi:10.7498/aps.73.20241063
Abstract +
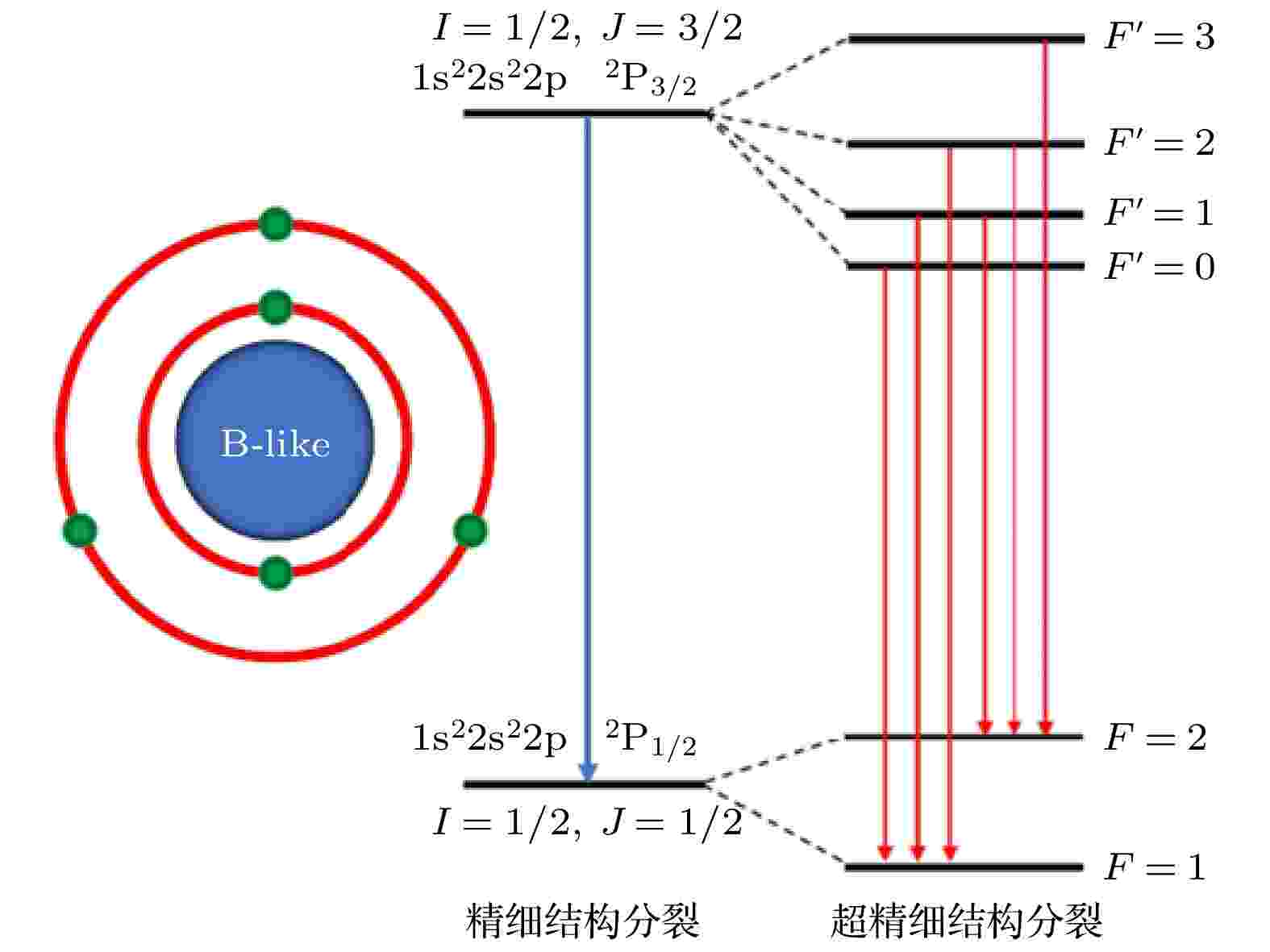
2024, 73 (20): 203102.
doi:10.7498/aps.73.20241190
Abstract +
The precise measurement of the fine structure and radiative transition properties of highly charged ions (HCI) is essential for testing fundamental physical models, including strong-field quantum electrodynamics (QED) effects, electron correlation effects, relativistic effects, and nuclear effects. These measurements also provide critical atomic physics parameters for astrophysics and fusion plasma physics. Compared with the extensively studied hydrogen-like and lithium-like ion systems, boron-like ions exhibit significant contributions in terms of relativistic and QED effects in their fine structure forbidden transitions. High-precision experimental measurements and theoretical calculations of these systems provide important avenues for further testing fundamental physical models in multi-electron systems. Additionally, boron-like ions are considered promising candidates for HCI optical clocks. This paper presents the latest advancements in experimental and theoretical research on the ground state2P3/2—2P1/2transition in boron-like ions, and summarizes the current understanding of their fine and hyperfine structures. It also discusses a proposed experimental setup for measuring the hyperfine splitting of boron-like ions by using an electron beam ion trap combined with high-resolution spectroscopy. This proposal aims to provide a reference for future experimental research on the hyperfine splitting of boron-like ions, to test the QED effects with higher precision, extract the radius of nuclear magnetization distribution, and validate relevant nuclear structure models.
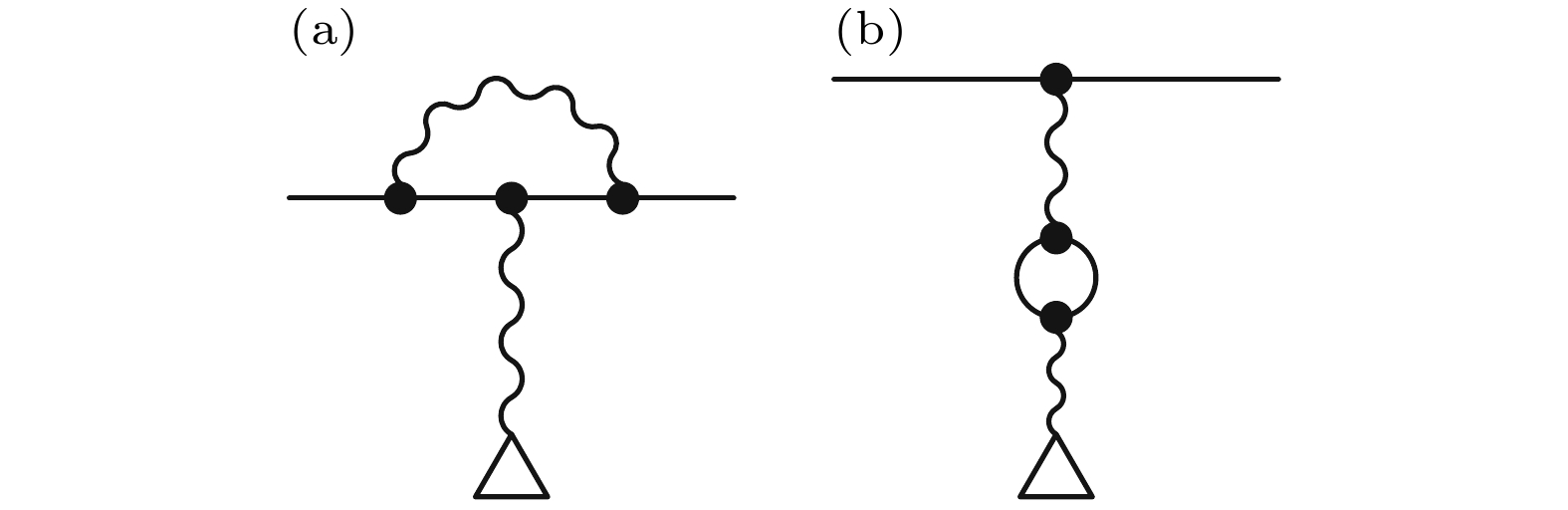
2024, 73 (20): 203103.
doi:10.7498/aps.73.20240683
Abstract +
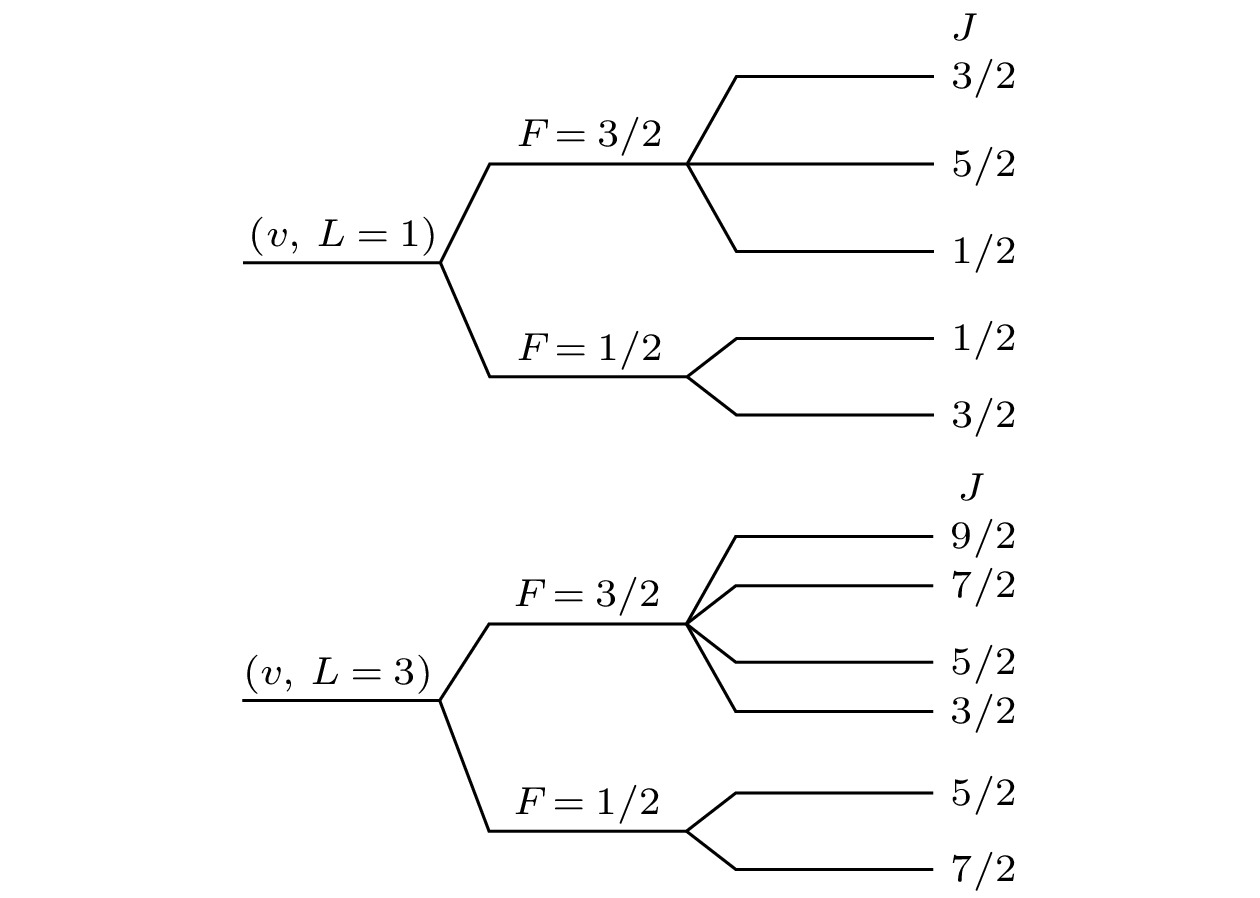
2024, 73 (20): 203104.
doi:10.7498/aps.73.20241101
Abstract +
The study of high-precision spectroscopy for hydrogen molecular ions enables the determination of fundamental constants, such as the proton-to-electron mass ratio, the deuteron-to-electron mass ratio, the Rydberg constant, and the charge radii of proton and deuteron. This can be accomplished through a combination of high precision experimental measurements and theoretical calculations. The spectroscopy of hydrogen molecular ions reveals abundant hyperfine splittings, necessitating not only an understanding of rovibrational transition frequencies but also a thorough grasp of hyperfine structure theory to extract meaningful physical information from the spectra. This article reviews the history of experiments and theories related to the spectroscopy of hydrogen molecular ions, with a particular focus on the theory of hyperfine structure. As far back as the second half of the last century, the hyperfine structure of hydrogen molecular ions was described by a comprehensive theory based on its leading-order term, known as the Breit-Pauli Hamiltonian. Thanks to the advancements in non-relativistic quantum electrodynamics (NRQED) at the beginning of this century, a systematic development of next-to-leading-order theory for hyperfine structure has been achieved and applied to $\text{H}_2^+$ and $\text{HD}^+$ in recent years, including the establishment of the $m\alpha^7\ln(\alpha)$ order correction. For the hyperfine structure of $\text{H}_2^+$, theoretical calculations show good agreement with experimental measurements after decades of work. However, for HD+, discrepancies have been observed between measurements and theoretical predictions that cannot be accounted for by the theoretical uncertainty in the non-logarithmic term of the $m\alpha^7$ order correction. To address this issue, additional experimental measurements are needed for mutual validation, as well as independent tests of the theory, particularly regarding the non-logarithmic term of the $m\alpha^7$ order correction.
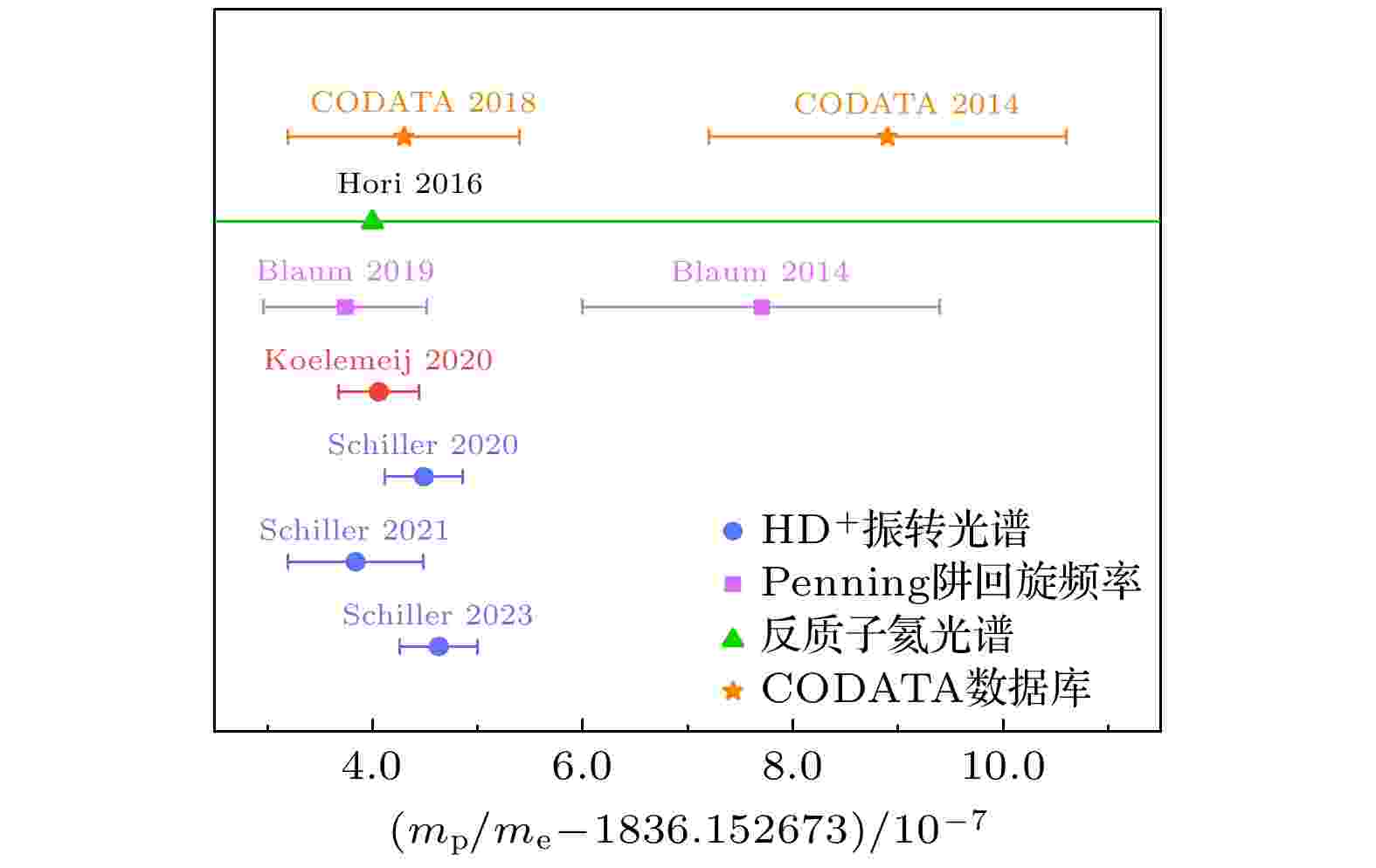
2024, 73 (20): 203301.
doi:10.7498/aps.73.20241064
Abstract +
A molecular hydrogen ion HD+, composed of a proton, a deuteron, and an electron, has a rich set of rovibrational transitions that can be theoretically calculated and experimentally measured precisely. Currently, the relative accuracy of the rovibrational transition frequencies of the HD+molecular ions has reached 10–12. By comparing experimental measurements with theoretical calculations of the HD+rovibrational spectrum, the precise determination of the proton-electron mass ratio, the testing of quantum electrodynamics(QED) theory, and the exploration of new physics beyond the standard model can be achieved. The experiment on HD+rovibrational spectrum has achieved the highest accuracy (20 ppt, 1 ppt = 10–12) in measuring proton-electron mass ratio. This ppaper comprehensively introduces the research status of HD+rovibrational spectroscopy, and details the experimental method of the high-precision rovibrational spectroscopic measurement based on the sympathetic cooling of HD+ions by laser-cooled Be+ions. In Section 2, the technologies of generating and trapping both Be+ions and HD+ions are introduced. Three methods of generating ions, including electron impact, laser ablation and photoionization, are also compared. In Section 3, we show the successful control of the kinetic energy of HD+molecular ions through the sympathetic cooling, and the importance of laser frequency stabilization for sympathetic cooling of HD+molecular ions. In Section 4, two methods of preparing internal states of HD+molecular ions, optical pumping and resonance enhanced threshold photoionization, are introduced. Both methods show the significant increase of population in the ground rovibrational state. In Section 5, we introduce two methods of determining the change in the number of HD+molecular ions, i.e. secular excitation and molecular dynamic simulation. Both methods combined with resonance enhanced multiphoton dissociation can detect the rovibrational transitions of HD+molecular ions. In Section 6, the experimental setup and process for the rovibrational spectrum of HD+molecular ions are given and the up-to-date results are shown. Finally, this paper summarizes the techniques used in HD+rovibrational spectroscopic measurements, and presents the prospects of potential spectroscopic technologies for further improving frequency measurement precision and developing the spectroscopic methods of different isotopic hydrogen molecular ions.
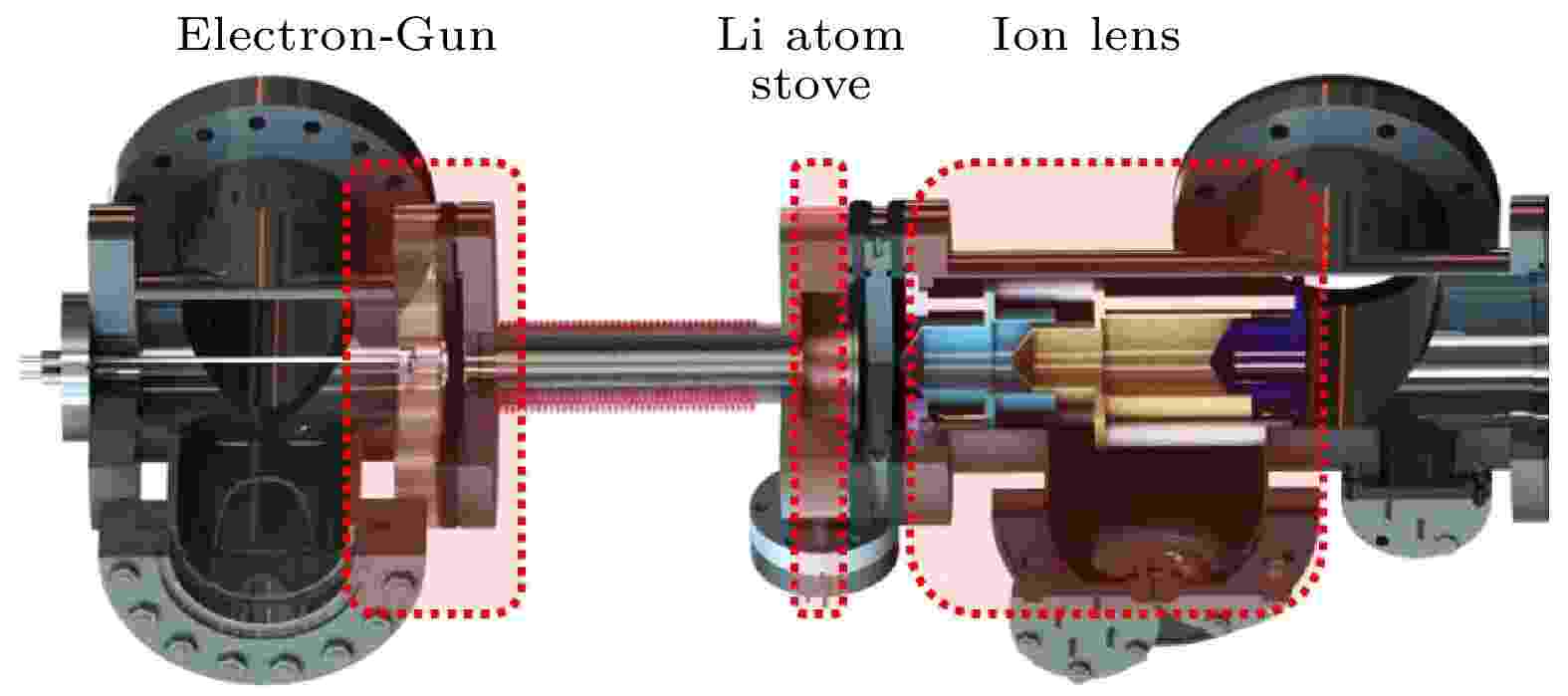
2024, 73 (20): 204203.
doi:10.7498/aps.73.20241128
Abstract +
Precision spectroscopy of lithium ions offers a unique research platform for exploring bound state quantum electrodynamics and investigating the structure of atomic nuclei. This paper overviews our recent efforts dedicated to the precision theoretical calculations and experimental measurements of the hyperfine splittings of6,7Li+ions in the $\,^3{\rm{S}}_1$ and $\,^3{\rm{P}}_J$ states. In our theoretical research, we utilize bound state quantum electrodynamics to calculate the hyperfine splitting of the $\,^3{\rm{S}}_1$ and $\,^3{\rm{P}}_J$ states with remarkable precision, achieving an accuracy on the order of $m\alpha^6$. Using Hylleraas basis sets, we first solve the non-relativistic Hamiltonian of the three-body system to derive high-precision energy and wave functions. Subsequently, we consider various orders of relativity and quantum electrodynamics corrections by using the perturbation method, with accuracy of the calculated hyperfine splitting reaching tens of kHz. In our experimental efforts, we developed a low-energy metastable lithium-ion source that provides a stable and continuous ion beam in the $\,^3{\rm{S}}_1$ state. Using this ion beam, we utilize the saturated fluorescence spectroscopy to enhance the precision of hyperfine structure splittings of7Li+in the $\,^3{\rm{S}}_1$ and $\,^3{\rm{P}}_J$ states to about 100 kHz. Furthermore, by utilizing the optical Ramsey method, we obtain the most precise values of the hyperfine splittings of6Li+, with the smallest uncertainty of about 10 kHz. By combining theoretical calculations and experimental measurements, our team have derived the Zemach radii of the6,7Li nuclei, revealing a significant discrepancy between the Zemach radius of6Li and the values predicted by the nuclear model. These findings elucidate the distinctive properties of the6Li nucleus, promote further investigations of atomic nuclei, and advance the precise spectroscopy of few-electron atoms and molecules.
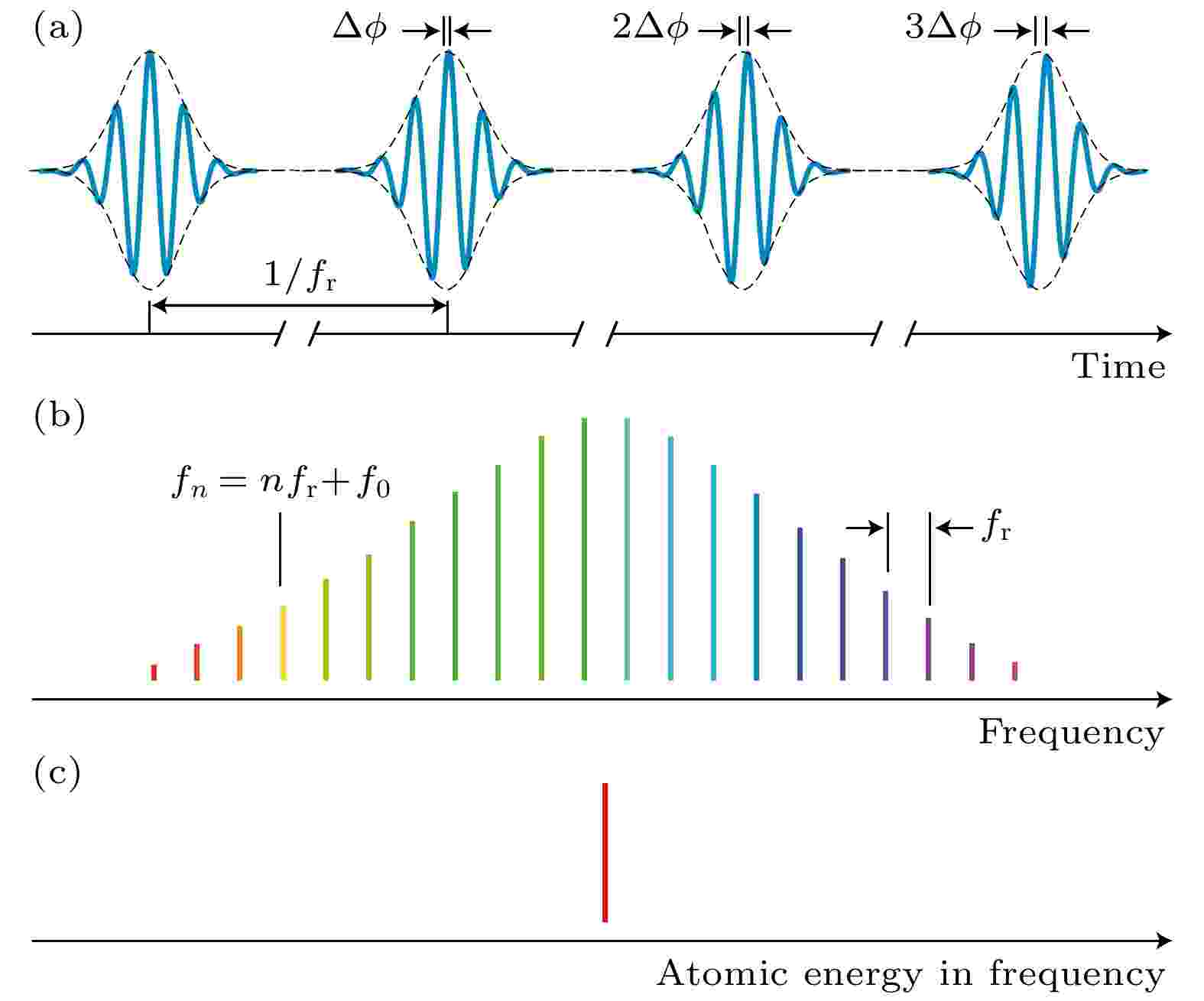
2024, 73 (20): 204205.
doi:10.7498/aps.73.20241231
Abstract +
REVIEW
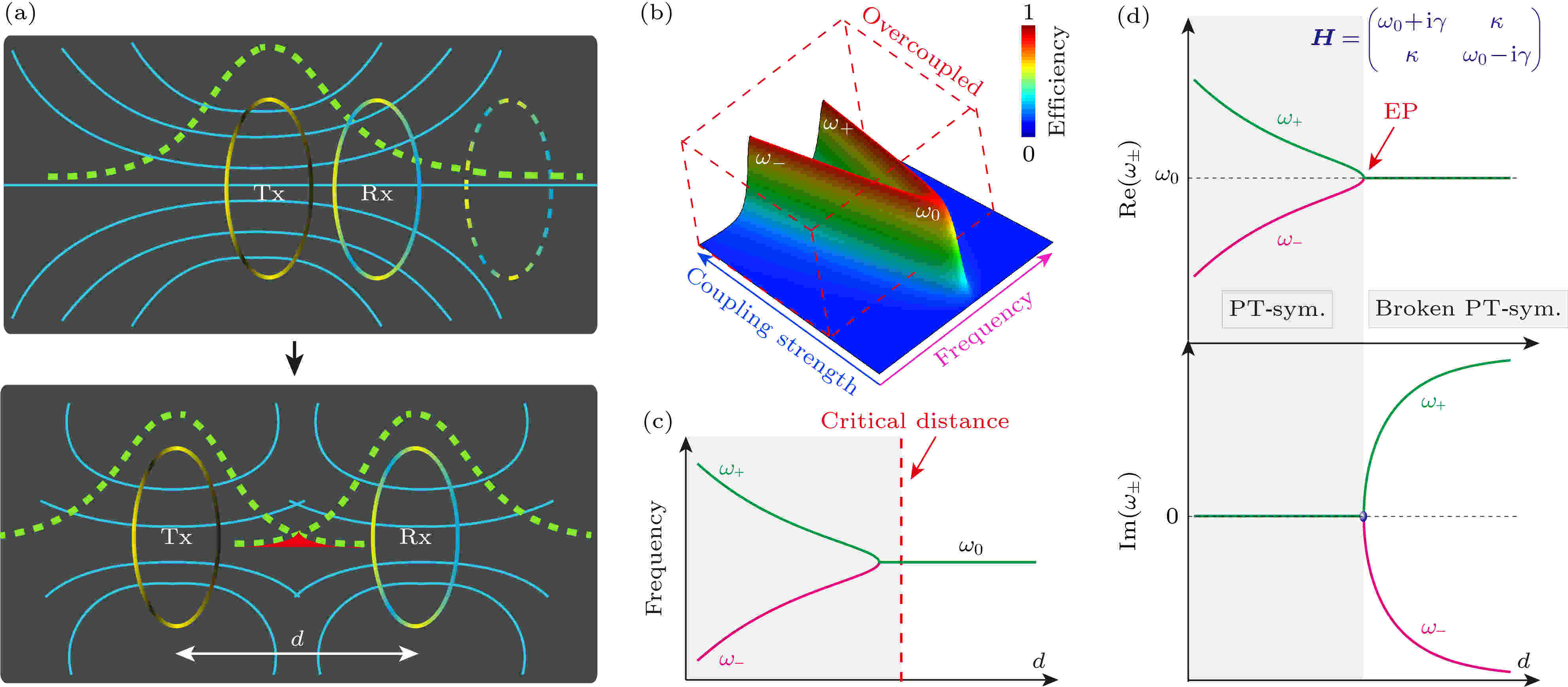
EDITOR'S SUGGESTION
2024, 73 (20): 201101.
doi:10.7498/aps.73.20241079
Abstract +
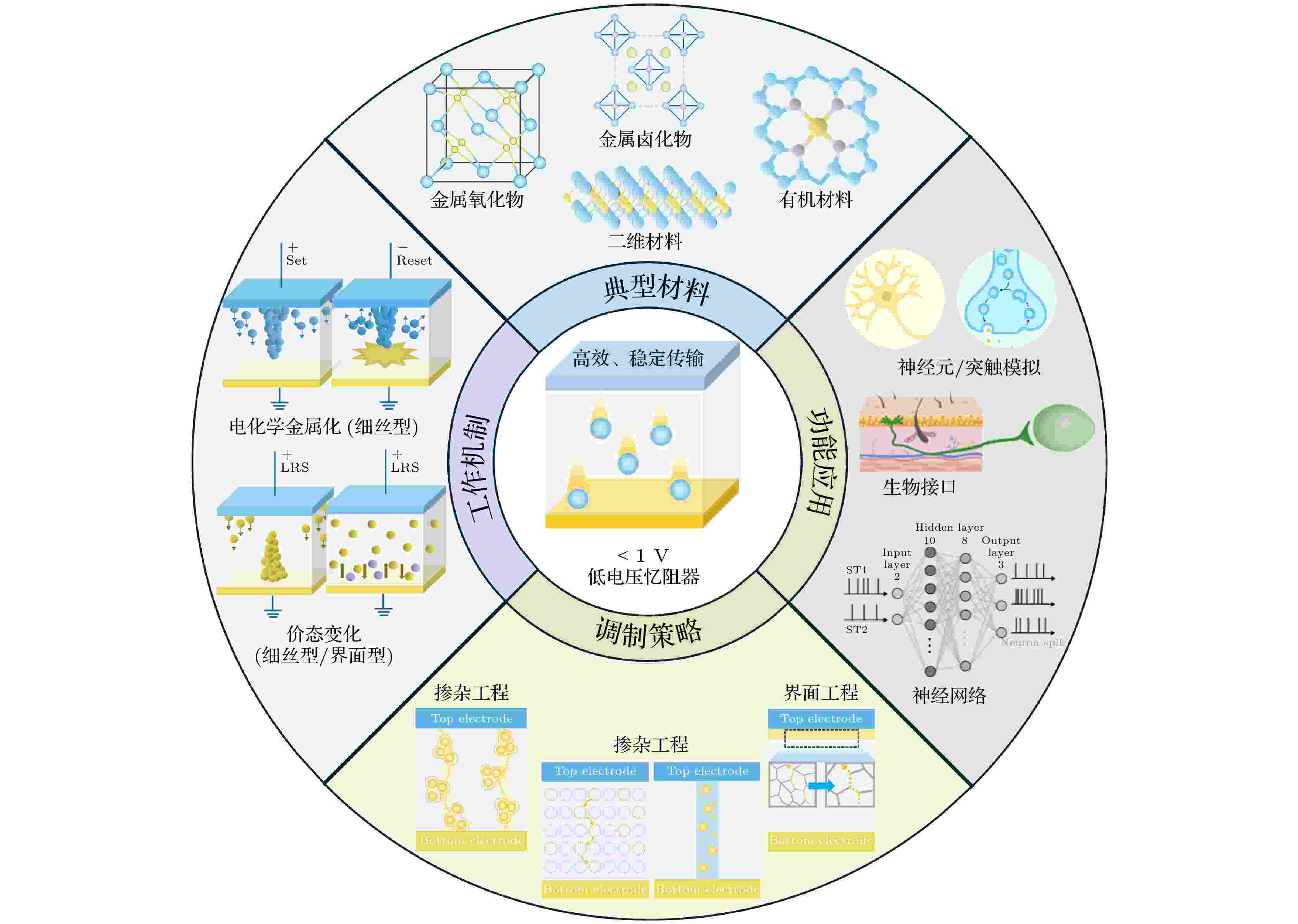
EDITOR'S SUGGESTION
2024, 73 (20): 207302.
doi:10.7498/aps.73.20241022
Abstract +
Memristors stand out as the most promising candidates for non-volatile memory and neuromorphic computing due to their unique properties. A crucial strategy for optimizing memristor performance lies in voltage modulation, which is essential for achieving ultra-low power consumption in the nanowatt range and ultra-low energy operation below the femtojoule level. This capability is pivotal in overcoming the power consumption barrier and addressing the computational bottlenecks anticipated in the post-Moore era. However, for brain-inspired computing architectures utilizing high-density integrated memristor arrays, key device stability parameters must be considered, including the on/off ratio, high-speed response, retention time, and durability. Achieving efficient and stable ion/electron transport under low electric fields to develop low-voltage, high-performance memristors operating below 1 V is critical for advancing energy-efficient neuromorphic computing systems. This review provides a comprehensive overview of recent advancements in low-voltage memristors for neuromorphic computing. Firstly, it elucidates the mechanisms that control the operation of low-voltage memristor, such as electrochemical metallization and anion migration. These mechanisms play a pivotal role in determining the overall performance and reliability of memristors under low-voltage conditions. Secondly, the review then systematically examines the advantages of various material systems employed in low-voltage memristors, including transition metal oxides, two-dimensional materials, and organic materials. Each material system has distinct benefits, such as low ion activation energy, and appropriate defect density, which are critical for optimizing memristor performance at low operating voltages. Thirdly, the review consolidates the strategies for implementing low-voltage memristors through advanced materials engineering, doping engineering, and interface engineering. Moreover, the potential applications of low-voltage memristors in neuromorphic function simulation and neuromorphic computing are discussed. Finally, the current problems of low-voltage memristors are discussed, especially the stability issues and limited application scenarios. Future research directions are proposed, focusing on exploring new material systems and physical mechanisms that could be integrated into device design to achieve higher-performance low-voltage memristors.
DATA PAPERS
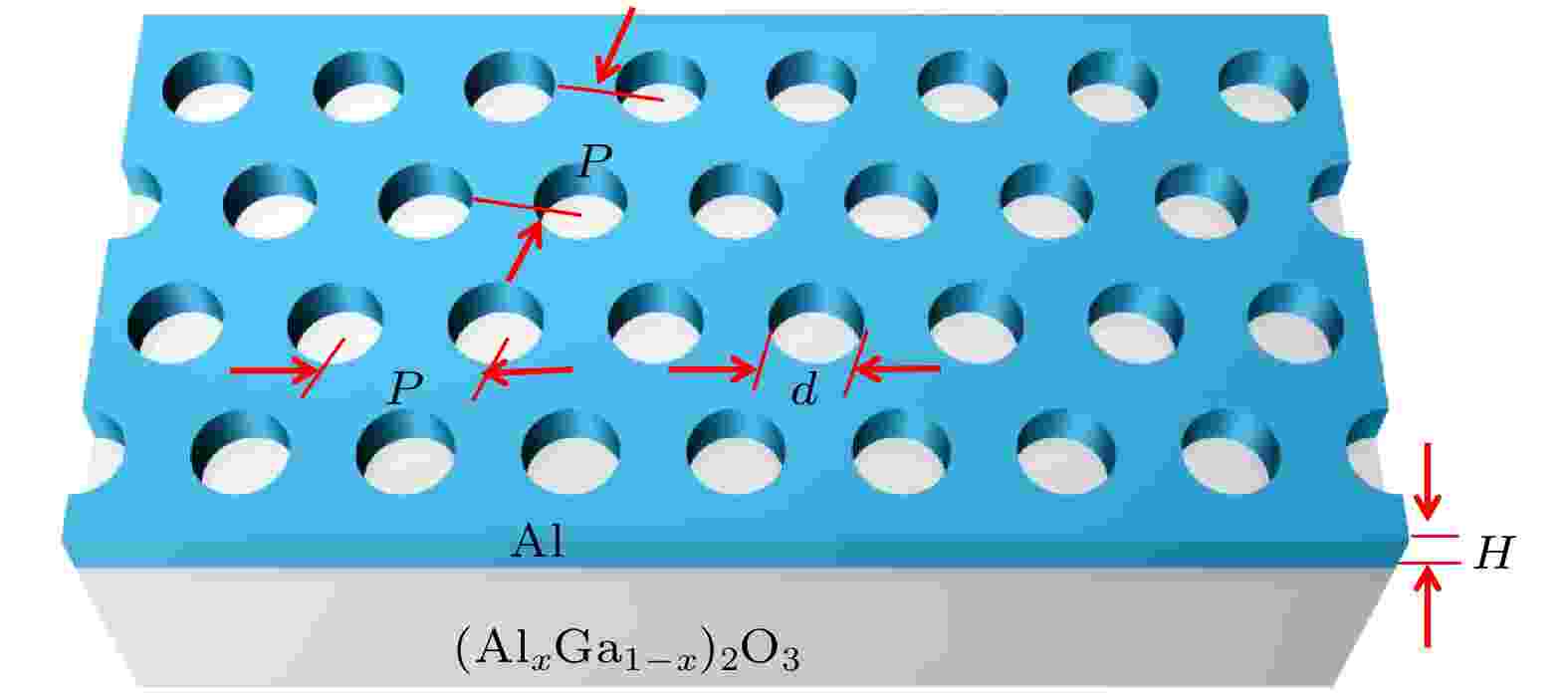
2024, 73 (20): 207801.
doi:10.7498/aps.73.20240928
Abstract +
GENERAL
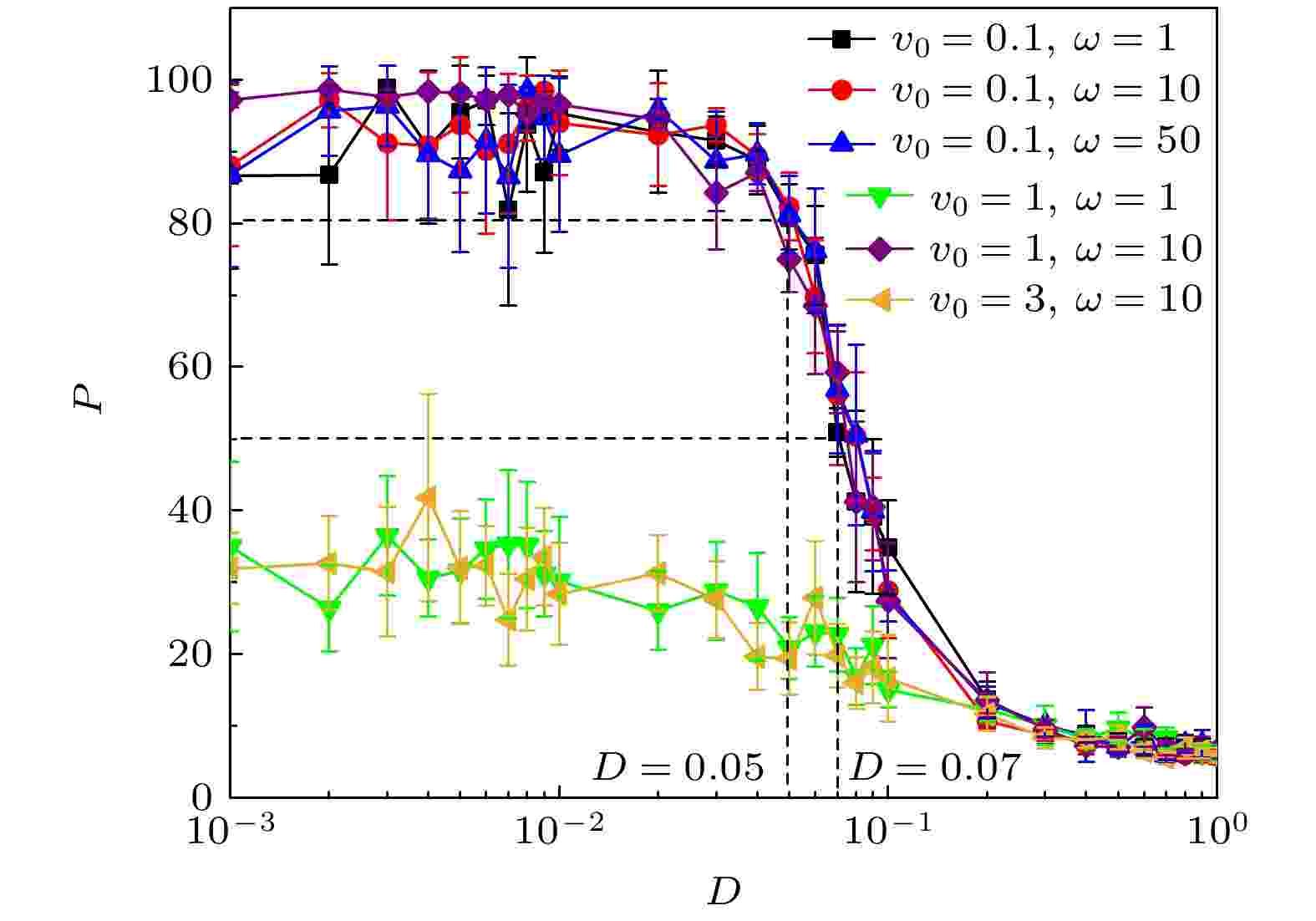
EDITOR'S SUGGESTION
2024, 73 (20): 200201.
doi:10.7498/aps.73.20240686
Abstract +
In recent years, the study of active particles has become one of the important topics concerned by researchers in many fields, among which the phase separation of active chiral particles has important theoretical and practical significance. In this paper, the phase separation of binary mixed systems composed of active chiral particles with different diffusion coefficients is studied by Langevin dynamics. A smaller relative diffusion coefficient is conducive to the formation of large clusters and the separation of “cold” particles, while a larger relative diffusion coefficient will weaken the separation effect. Due to the influence of particle characteristics (self-driven velocity, self-rotational angular velocity) and relative diffusion coefficient on the collision between particles, if one wants the “cold” and “hot” particles to reach phase separation, increasing (or reducing) the self-driven velocity and self-rotational angular velocity cannot be synchronous, and the relative rate of change of self-driven velocity is smaller than that of the self-rotational angular velocity. By analyzing the changes of the effective diffusion coefficient of “cold” particles, the phenomenon of phase separation in the system can be better explained. A smaller effective diffusion coefficient means that the “cold” particles will aggregate into larger clusters, and the system may exhibit phase separation. However, when the effective diffusion coefficient is larger, the diffusion of “cold” particles is stronger and the “cold” particles will not form large clusters, which means that the system cannot aggregate into phase separation. In addition, with the filling rate of particle increasing, the proportion curve of the number of cold particles in maximum cold particle cluster undergoes a non-monotonic change, specifically, it first increases and then decreases. Each curve has an optimal filling rate but its width is different .With the increase of the relative diffusion coefficient and self-driven velocity, the width of the optimal filling rate of the proportion curve will become narrower and shift toward the right.
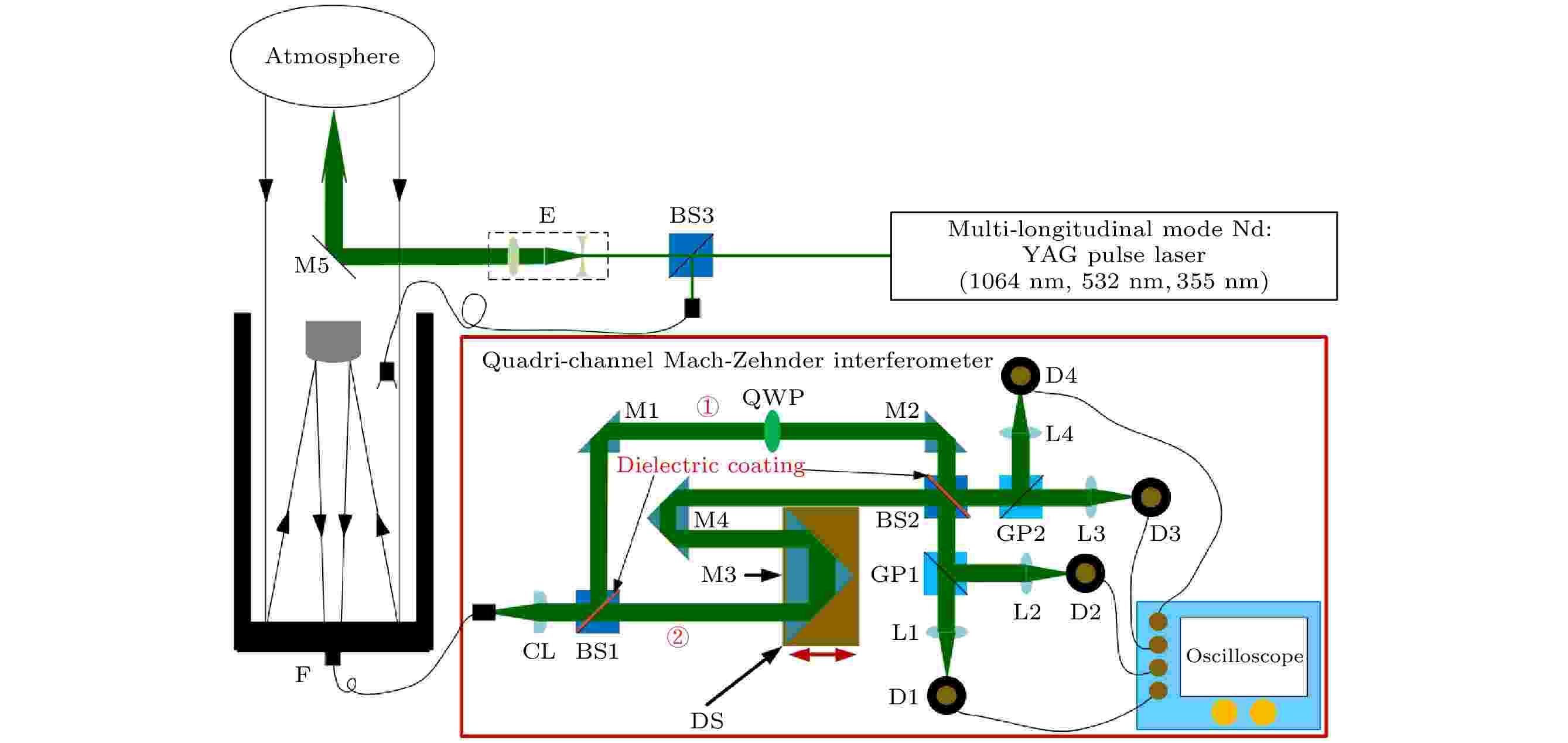
2024, 73 (20): 200701.
doi:10.7498/aps.73.20240949
Abstract +
ATOMIC AND MOLECULAR PHYSICS
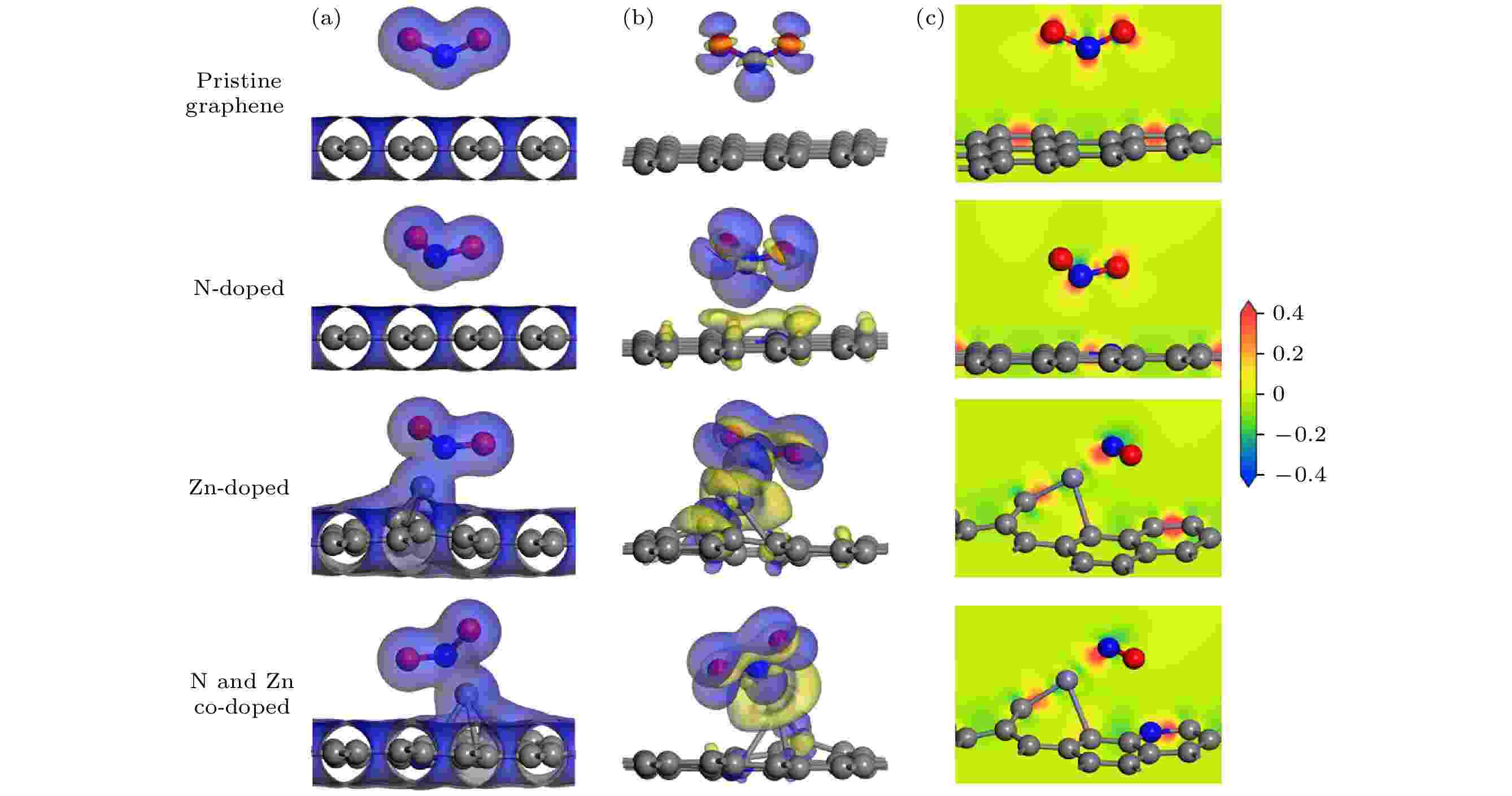
2024, 73 (20): 203101.
doi:10.7498/aps.73.20240992
Abstract +
In order to study the adsorption of NO2on pristine graphene and doped graphene (N-doped, Zn-doped, and N-Zn co-doped), we simulate the adsorption process by applying the first-principles plane-wave ultrasoft pseudopotentials of the density-functional theory in this work. The adsorption energy, Mulliken distribution, differential charge density, density of states, and optical properties of NO2molecules adsorbed on the graphene surface are calculated. The results show that the doped graphene surface exhibits higher sensitivity to the adsorption of NO2compared with the pristine graphene surface, and the order of adsorption energy is as follows: N-Zn co-doped surface > Zn-doped surface > N-doped surface > pristine surface. Pristine graphene surface and N-doped graphene surface have weak interactions with and physical adsorption of NO2. Zn-doped graphene surfac and N-Zn co-doped graphene surface form chemical bonds with NO2and are chemisorbed. In the visible range, among the three doping modes, the N-Zn co-doped surface is the most effective for improving the optical properties of graphene, with the peak absorption and reflection coefficients improved by about 1.12 and 3.42 times, respectively, compared with pristine graphene. The N-Zn co-doped graphene not only enhances the interaction between the surface and NO2, but also improves the optical properties of the material, which provides theoretical support and experimental guidance for NO2gas detection and sensing based on graphene substrate.
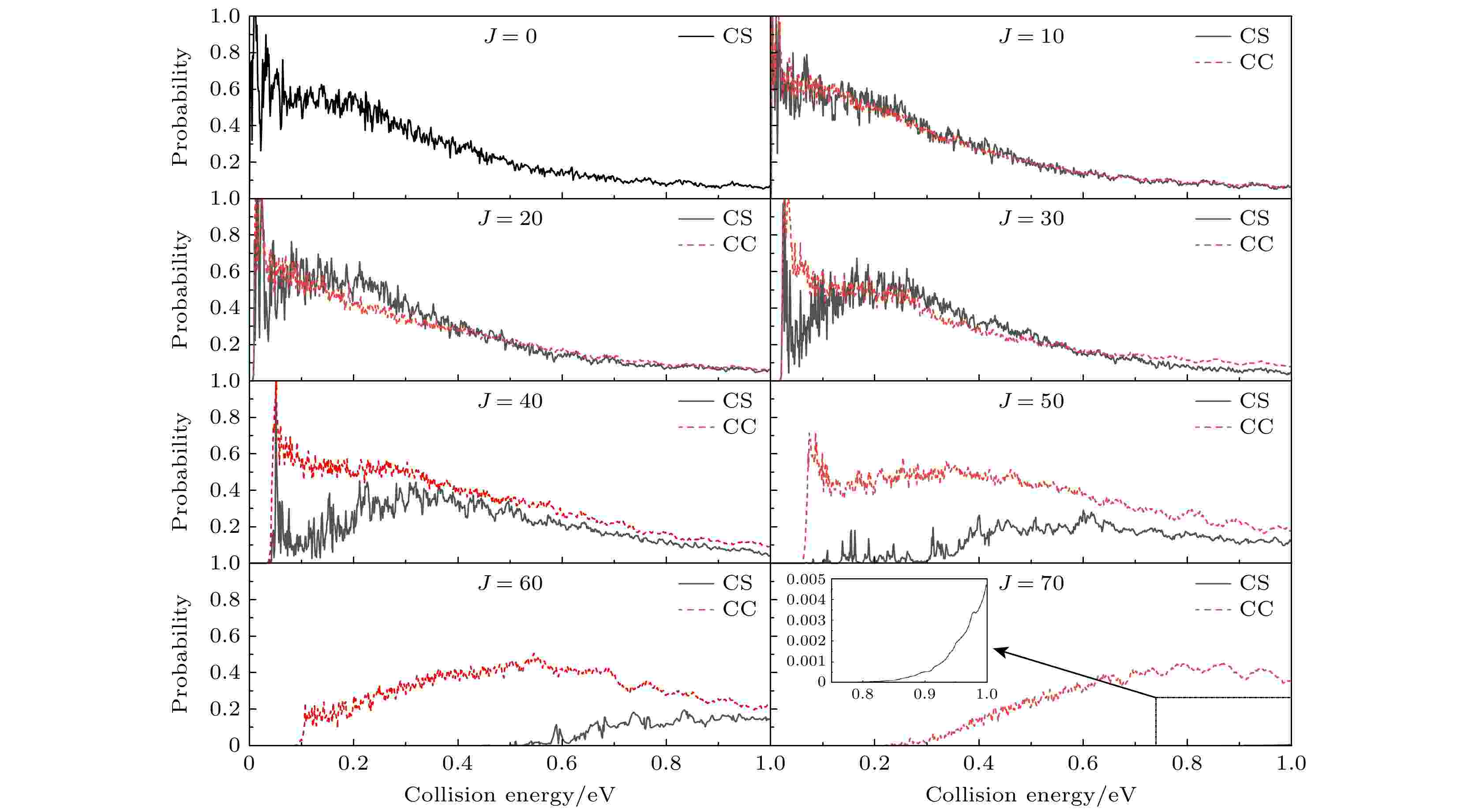
2024, 73 (20): 203401.
doi:10.7498/aps.73.20240859
Abstract +
Initial state-selected and energy-resolved reaction probabilities, integral cross sections(ICSs), and thermal rate constants of the $ \text{H}{(}^{2}\text{S})+S\text{iH}({\text{X}}^{2}\Pi; \nu = 0\text{ },j = 0)\to \text{Si}{(}^{1}\text{D})+{\text{H}}_{2}({\text{X}}^{1} \Sigma_{g}^{+}) $ reaction are calculated within the coupled state(CS) approximation and accurate calculation with full Coriolis coupling(CC) by a time-dependent wave packet propagation method (Chebyshev wave packet method). Therefore, a new ab initio global potential energy surface (PES) of the electronic ground state (11A′) of the system, which was recently reported by Li et al. [
Phys. Chem. Chem. Phys.2022
247759
], is employed. The contributions of all partial waves to the total angular momentumJ= 80 for CS approximation andJ= 90 for CC calculation are considered to obtain the converged ICSs in a collision energy range of 1.0 ×10–3-1.0 eV. The calculated probabilities and ICSs display a decreasing trend with the increase of the collision energy and show an oscillatory structure due to the SiH2well on the reaction path. The neglect of CC effect will lead to underestimation of the ICS and the rate constant due to the formation of an SiH2complex supported by the stationary points of the SiH2(11A′) PES. In addition, the results of the exact calculation including CC effect are compared with those calculated in the CS approximation. For the reaction probability, CC and CS calculations change with similar tends, shown by their observations at small total angular momentumJ= 10, 20 and 30, and the CC results are larger than the CS results almost in the whole considered energy range at large total angular momentumJ= 40, 50, 60 and 70. The gap between CS and CC probability get more pronounced with increasing ofJ, which reveals that Coriolis coupling effects become more and more important withJincreasing for the title reaction.Moreover, the exact quantum-wave calculations show that the thermal rate constant between 300 K and 1000 K for the title reaction shows a similar temperature independent behavior to that for the H + CH reaction, but the value of the rate constant for the H + SiH reaction is an order of magnitude larger than that for the H + CH reaction.
ELECTROMAGNETISM, OPTICS, ACOUSTICS, HEAT TRANSFER, CLASSICAL MECHANICS, AND FLUID DYNAMICS
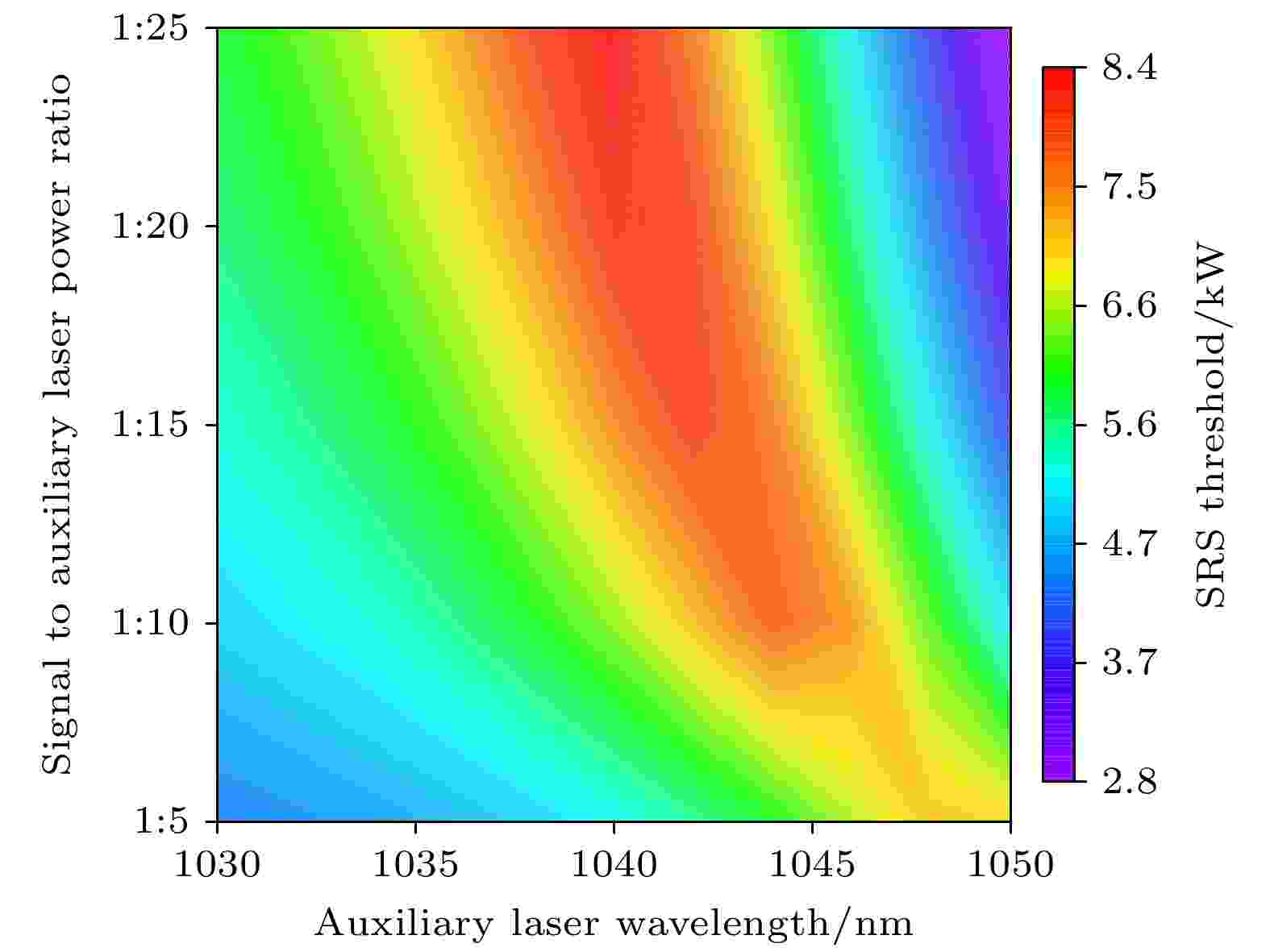
2024, 73 (20): 204201.
doi:10.7498/aps.73.20240895
Abstract +
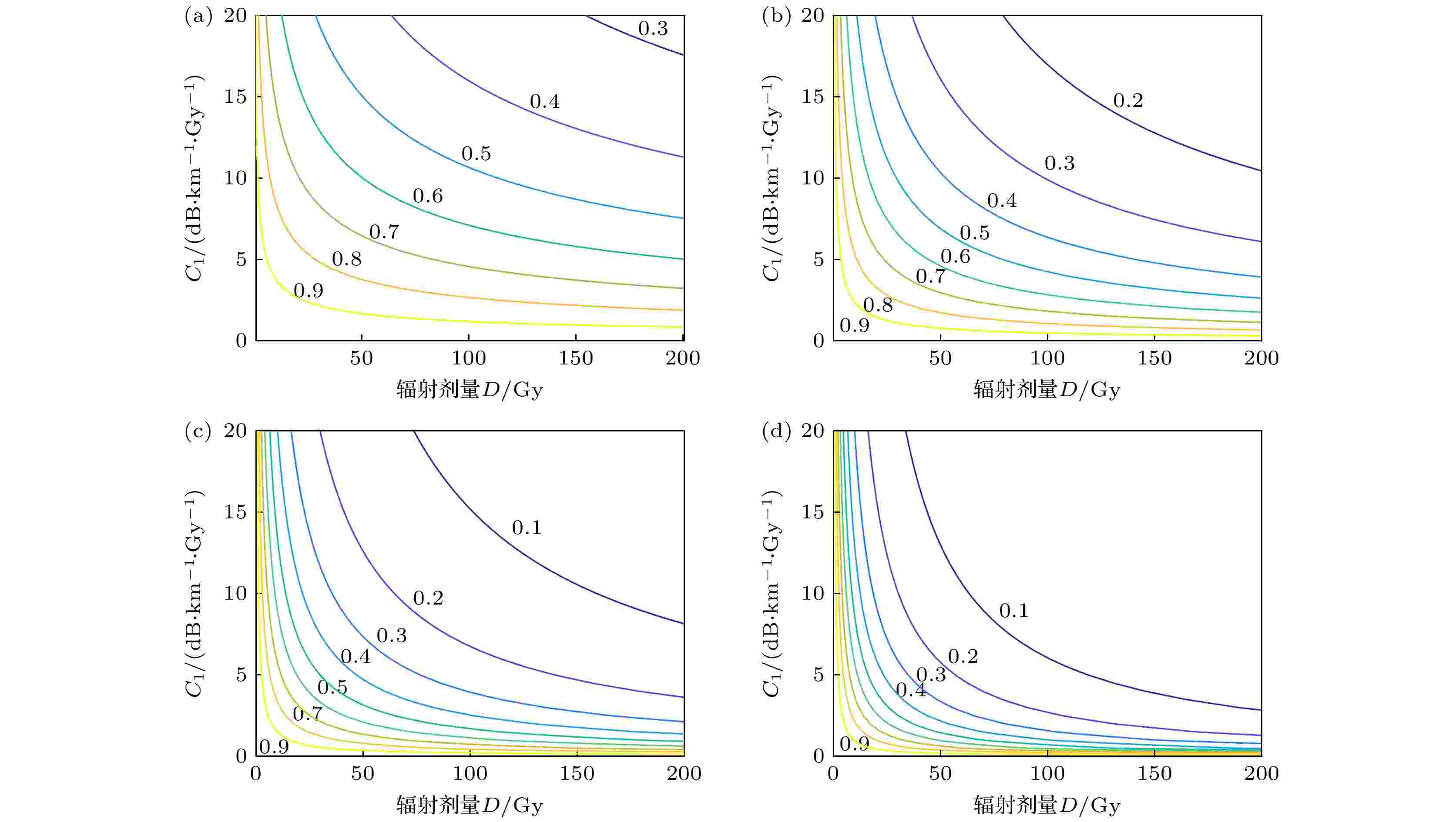
2024, 73 (20): 204202.
doi:10.7498/aps.73.20240816
Abstract +
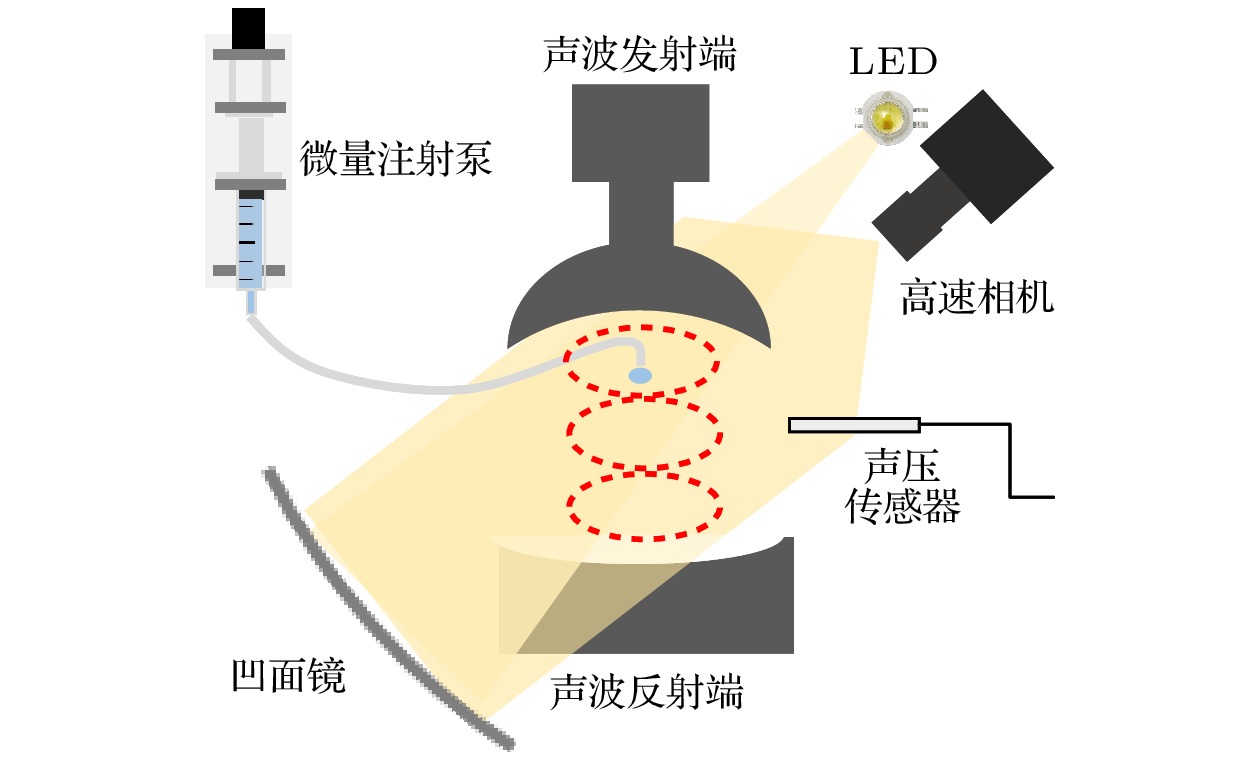
2024, 73 (20): 204204.
doi:10.7498/aps.73.20240965
Abstract +
The advancement of the theory of droplet stability in the acoustic field is of significant value in improving ultrasonic atomization and ultrasonic levitation technology. In this work, in order to reveal the detailed mechanism of acoustic droplet instability and give the instability criterion for easy application, the dynamics of droplet instability in standing wave acoustic field (19.8 kHz) is studied by combining practical experiment, theoretical derivation and numerical calculation. The acoustic instability of the droplet occurring near the wave nodes is mainly manifested in two typical modes: disk instability and edge-sharpening instability. The appearance of these two instability modes depends on the relative magnitude of the standing wave field strength. Specifically, with the gradual enhancement of the intensity of the standing wave field, the instability mode of the droplet will gradually change from disc instability to edge-sharpened instability.The droplets show obvious self-accelerating expansion in the equatorial plane in the instability process. The positive feedback between the droplet aspect ratio and the negative pressure of acoustic radiation at the equator of the droplet is the reason for the above self-accelerating behavior. The theoretical results obtained through deduction indicate that the amplitude of the negative acoustic radiation pressure at the droplet equator is proportional to the square of the droplet aspect ratio. The surface tension of the droplet is the main factor hindering its deformation, while the acoustic radiation suction at the equator is the main factor driving the deformation of the droplet. Based on this, the force equilibrium equation of the droplet interface is established, and the dimensionless criterion of acoustic droplet instability, i.e. the acoustic Weber numberWea, is derived. WhenWea≤1, the droplet interface stays in equilibrium, and whenWea>1, the equatorial acoustic suction is larger than the surface tension, and the droplet instability occurs, and the average error between the experimental results and the theoretical results is only 9%.
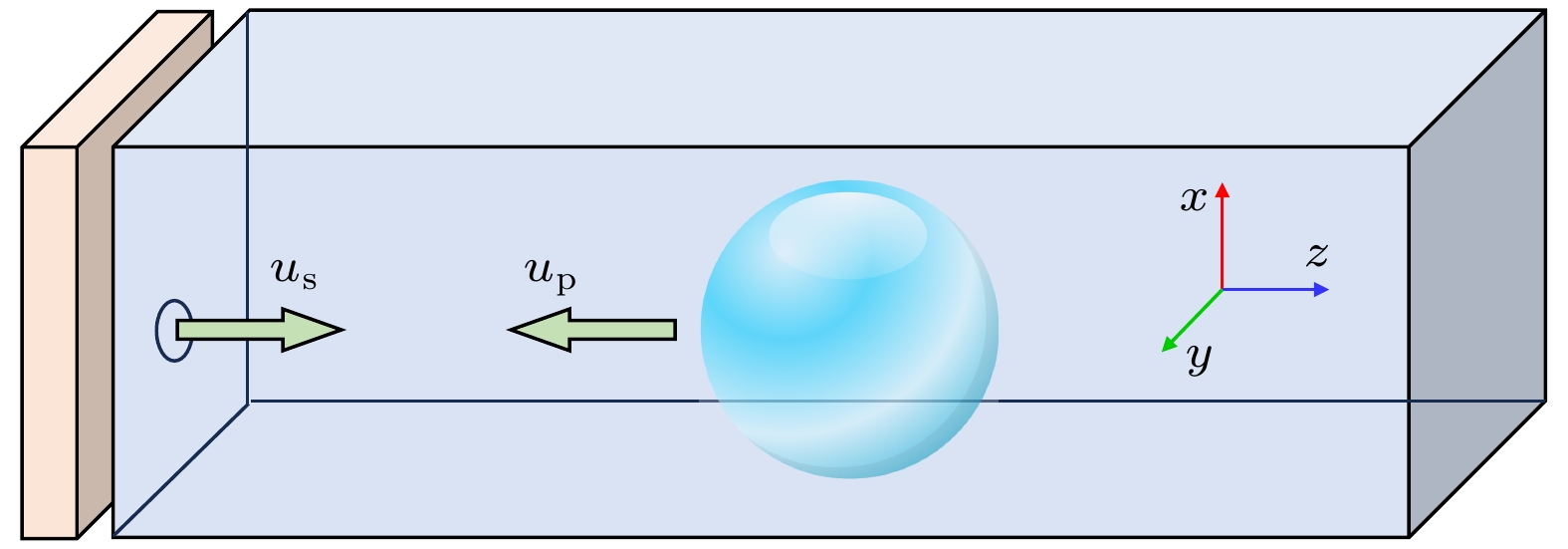
2024, 73 (20): 204701.
doi:10.7498/aps.73.20241105
Abstract +
This study employs molecular dynamics simulations to investigate the process of nanobubble gradual indentation and eventual collapse. The research primarily focuses on the mechanisms by which impact velocity and bubble size influence the dynamic characteristics of nanobubble collapse. The results indicate that nanobubble collapse generally proceeds through three stages. Initially, there is a compression phase of water molecules surrounding the bubble, followed by a phase where the shock wave disrupts the stable structure of the liquid film, and finally, the complete collapse of the bubble. At higher impact velocities, smaller bubbles collapse more rapidly due to stronger shock effects. Post-collapse, a high-speed jet forms a protrusion on the right end of the velocity contour. The degree of protrusion increases with bubble size and impact velocity. Water molecules converge towards the bubble center, forming vortex structures above and below the bubble, effectively enhancing internal mass transfer. As bubble size and impact velocity increase, the density around the bubble gradually rises, reaching approximately 1.5 g/cm³ in localized areas upon complete collapse. When the bubble system decays to half its original size, a water hammer effect occurs. This effect becomes more pronounced with increasing bubble size and impact velocity. For a nanobubble structure withup= 3.0 km/s andD= 10 nm, the local pressure formed by the water hammer impact of the jet after collapse can reach 30 GPa.
CONDENSED MATTER: STRUCTURAL, MECHANICAL, AND THERMAL PROPERTIES
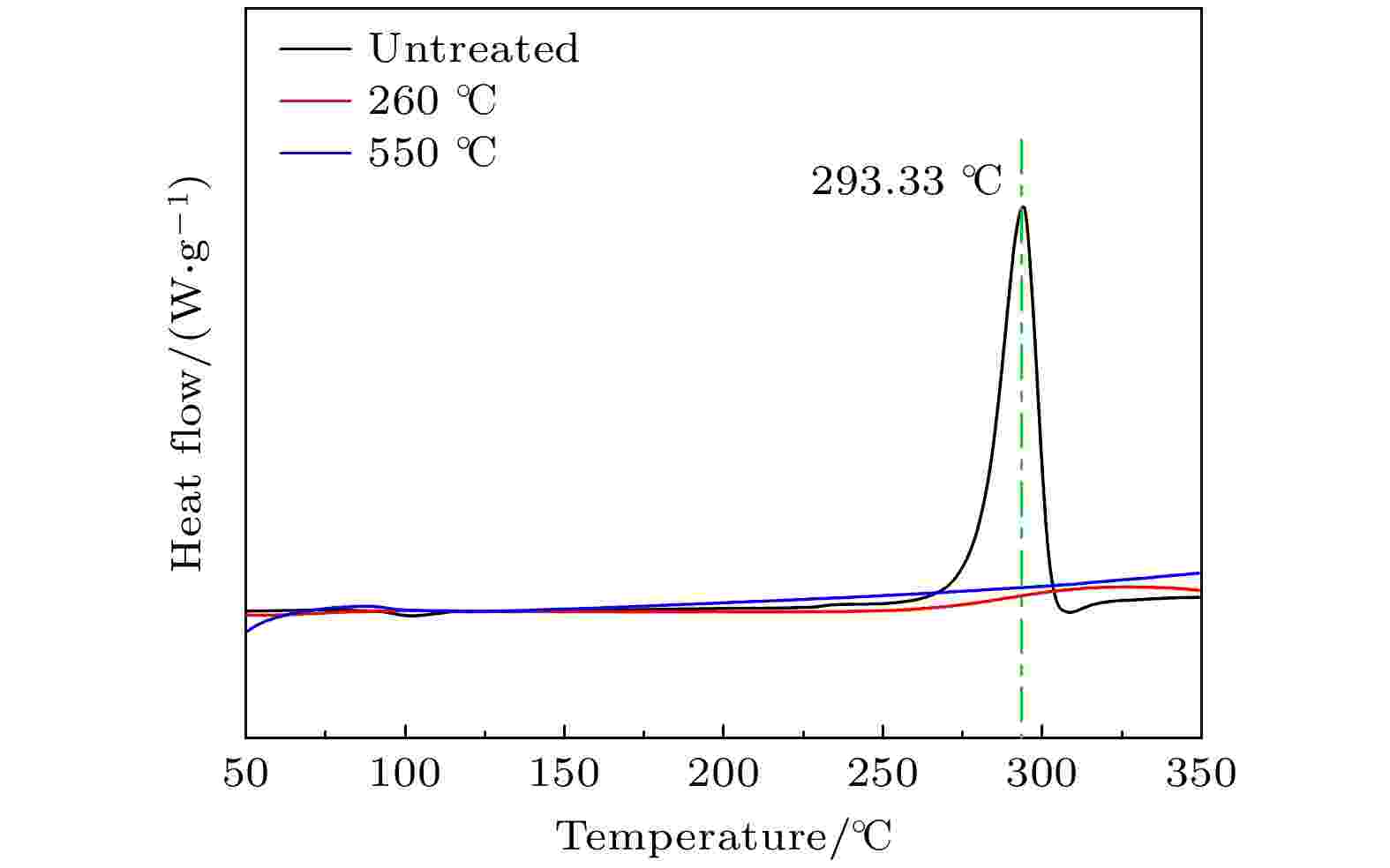
2024, 73 (20): 206801.
doi:10.7498/aps.73.20241006
Abstract +
Most of existing piezoelectric polymers have low glass transition temperatures, so they can only operate at lower temperatures (<150 ℃). Once the operating temperature is exceeded, the piezoelectric performance of the device rapidly decreases. At higher temperatures, dense chain motion can interfere with the orientation of dipoles, thus limiting the development of polymer based high-temperature piezoelectric sensors. High-temperature piezoelectric sensor devices are entirely made of inorganic materials, however, inorganic materials are rigid and can only work under small strains. Therefore, enhancing the temperature resistance of piezoelectric polmers and constructing piezoelectric asymmetric structure are the key to fabricating flexible high-temperature resistant piezoelectric/pyroelectric dual functional sensors. In this study, polyacrylonitrile (PAN) nanofiber film is prepared by electrospinning, and then subjected to heat treatment through programmed temperature control. The effects of the different heat-treatment temperatures on the mechanical and electrical performance of PAN nanofiber film are studied systematically, and the results show that PAN high temperature resistant flexible nanofiber film sensors can be used in high temperature environments (>500 ℃). Its output performance is improved with the increase of heat treatment temperature (<260 ℃) and then basically remains unchanged in a temperature range of 260–450 ℃. Finally, the output performance decreases at temperatures higher than 450 ℃. When the heat treatment temperature reaches 260 ℃, the output voltage increases to 10.08 V, and current reaches 2.89 μA. Compared with those of the untreated PAN membranes , its output voltage and current are increase by 3.54 times and 2.83 times, respectively. At the same time, the output of the PAN high temperature resistant flexible nanofiber film sensors is almost unchanged in the high-temperature environments. This is the first time that the pyroelectric effect has been observed in heat-treated PAN nanofiber films and both the open-circuit voltage and short-circuit current have been shown to increase with temperature gradient increasing. Besides, the PAN nanofiber film sensors have durability of more than 5000 cycles at room temperature(25 ℃) even at high temperature (400 ℃). Overall, good flexible, high-temperature resistance, and bifunctional sensing ability make PAN flexible nanofiber film sensors expected to be widely used in high temperature environments such as fire safety, aerospace and other harsh environment.
CONDENSED MATTER: ELECTRONIC STRUCTURE, ELECTRICAL, MAGNETIC, AND OPTICAL PROPERTIES
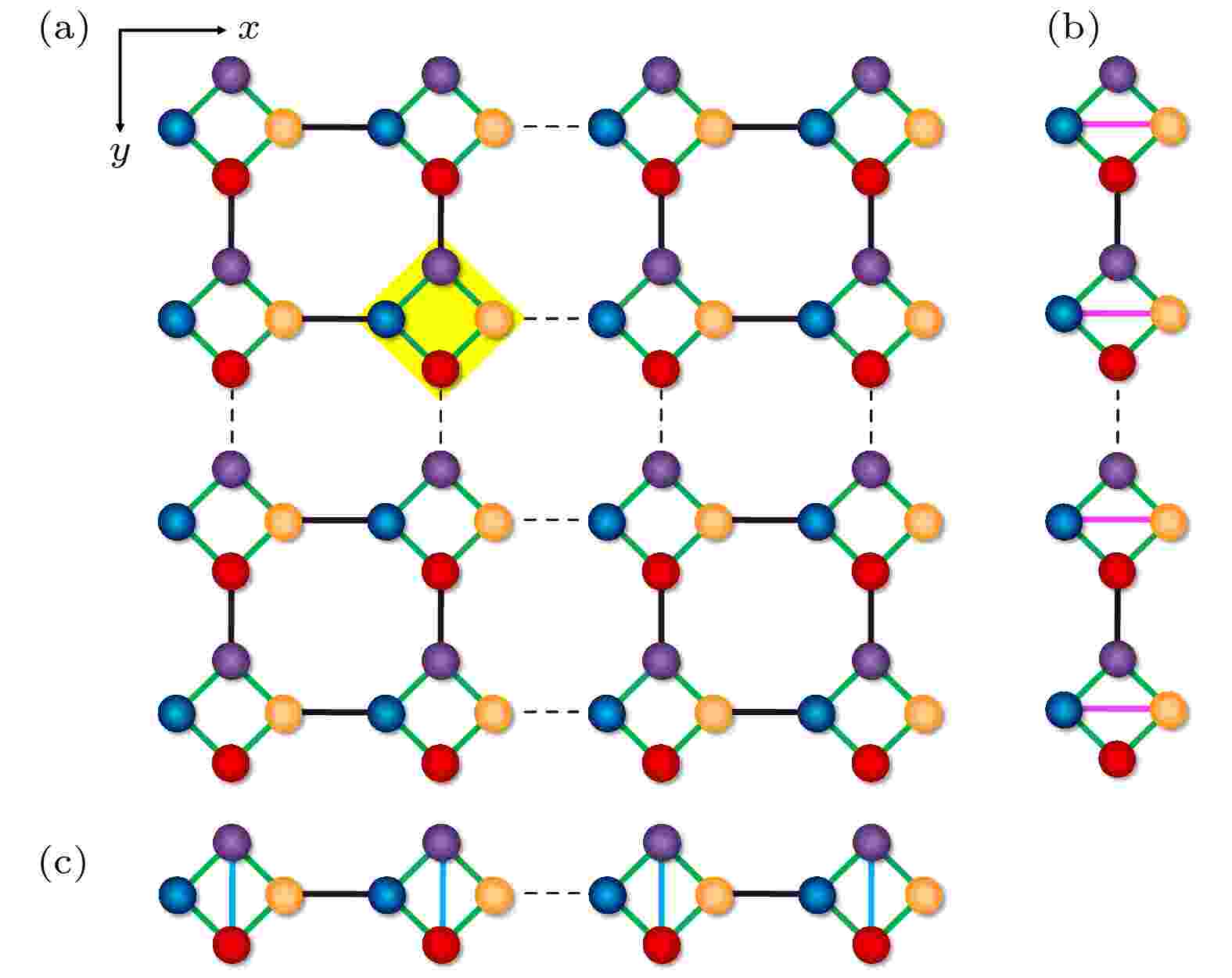
2024, 73 (20): 207301.
doi:10.7498/aps.73.20240871
Abstract +
This paper investigates the regulatory effect of non-Hermitian mechanisms on energy spectra and edge states by applying a single- or double-layer imaginary potential with parity-time (PT) symmetry to both sides of the T-graphene ribbon. The findings indicate that the type of imaginary potential applied has a significant modulation effect on the energy band structure and localization of the system. Specifically, when an imaginary potential is applied to the outermost monolayer lattice point of the ribbon, the energy of the edge state appears in the imaginary part. For its probability density distribution, its locality changes from both-sided to one-sided locality, and becomes stronger with the increase of imaginary potential. Additionally, the PT symmetry phase transition occurs in the topologically trivial region. Notably, as the imaginary potential reaches a critical value, new imaginary-energy edge state emerges within the bulk state energy gap and also shows the phenomenon that the localization is on one side of the system. Furthermore, when double-layer imaginary potentials are applied, two different edge states will appear in the system. The first type appears in the top band and the bottom band, localized on one side of the system. The second type emerges in the middle of the second energy band and the third energy band, displaying relatively weak localization and not penetrating the energy gap. This work contributes to understanding the regulatory effect of the edge imaginary potential of PT symmetry on the physical properties of T-graphene structures.
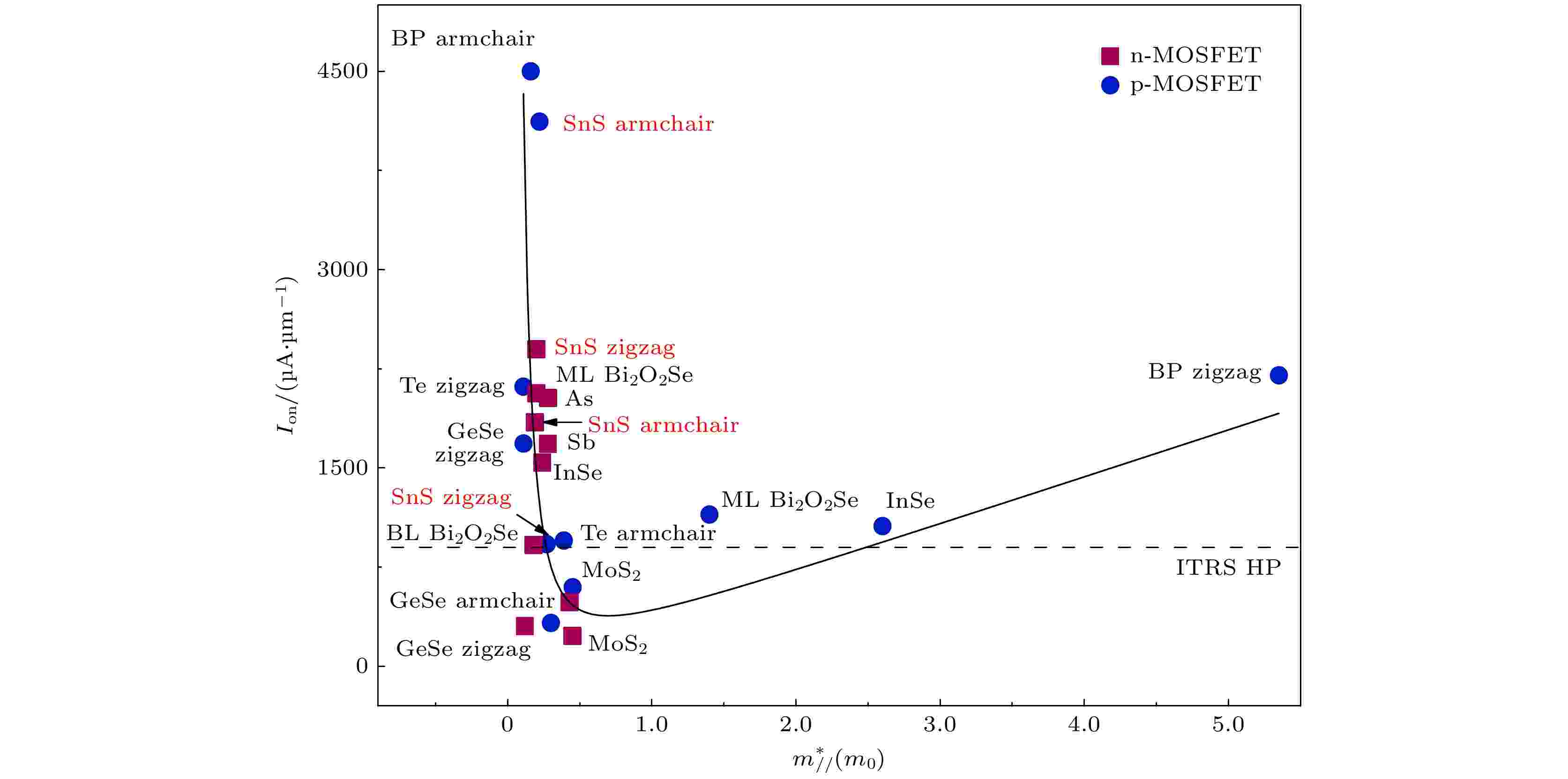
EDITOR'S SUGGESTION
2024, 73 (20): 207304.
doi:10.7498/aps.73.20241004
Abstract +
COVER ARTICLE
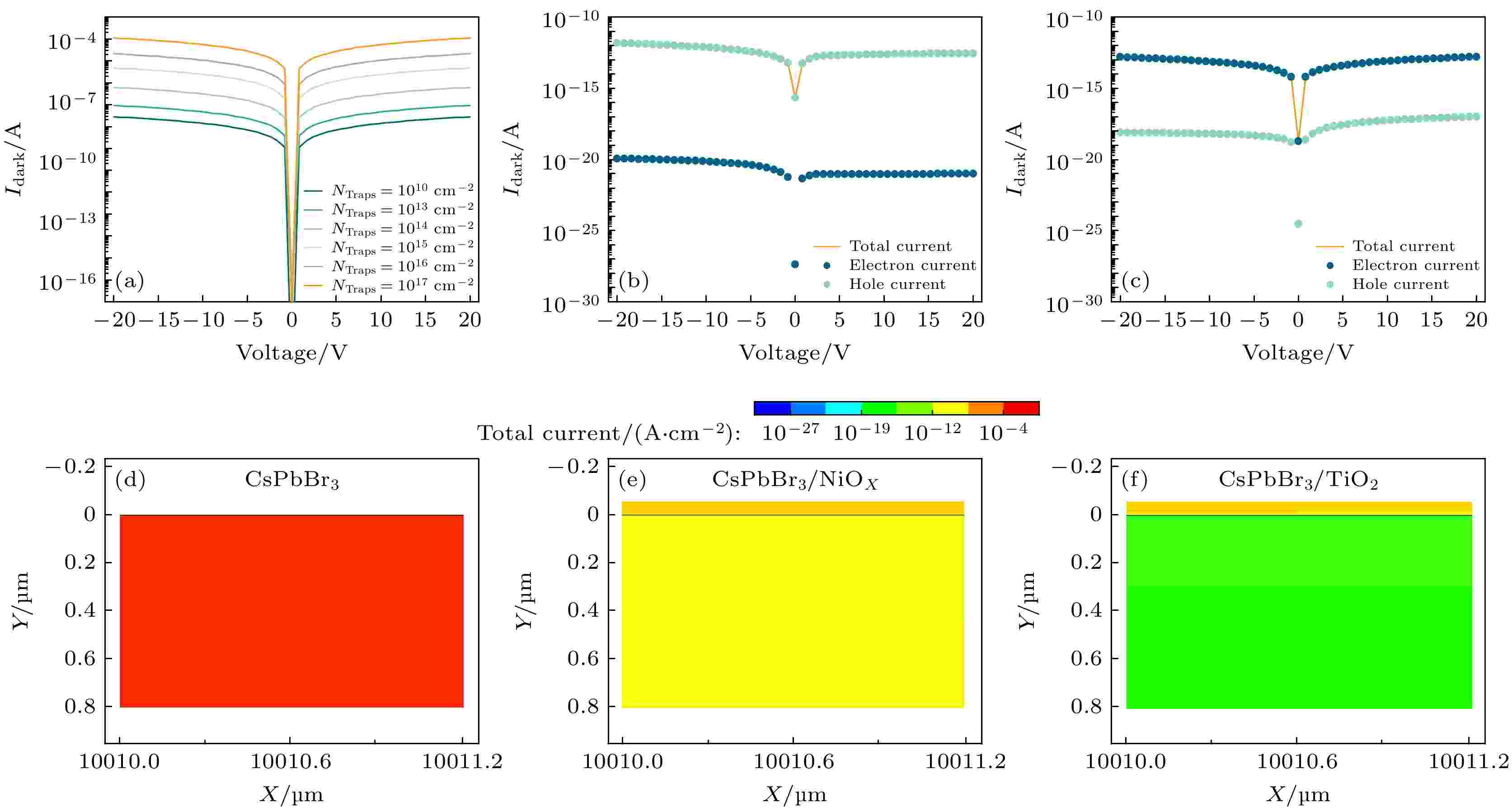
COVER ARTICLE
2024, 73 (20): 208501.
doi:10.7498/aps.73.20241075
Abstract +
Halide perovskites exhibit excellent electrical and optical properties and are ideal candidates for active layers in optoelectronic devices, especially in the field of high-performance photodetection, where they demonstrate a competitive advantage in terms of development prospects. Among them, the all-inorganic perovskite CsPbBr3has received widespread attention due to its better environmental stability. It is demonstrated in this work that a vertical MSM-type CsPbBr3thin-film photodetector has characteristics of fast response time and ultra-low dark current. The use of a vertical structure can reduce the transit distance of photo carriers, enabling the device to achieve a fast response time of 63 μs, which is two orders of magnitude higher than the traditional planar MSM-type photodetectors with a response time of 10 ms. Then, by spinning a charge transport layer between the p-type CsPbBr3and Ag electrodes, effective separation of photocarriers at the interface is realized and physical passivation between the perovskite and metal electrodes is also achieved. Due to the superior surface quality of the spun TiO2film compared with the NiOxfilm, and through Sentaurus TCAD simulations and bandgap analyses, with TiO2serving as the electron transport layer, it effectively inhibits the transmission of excess holes in p-type CsPbBr3. Consequently, the electron transport layer TiO2is more effective in reducing dark current than the hole transport layer NiOx, with a dark current magnitude of only –4.81×10–12A at a –1 V bias. Furthermore, this vertical MSM-type CsPbBr3thin-film photodetector also has a large linear dynamic range (122 dB), high detectivity (1.16×1012Jones), and good photo-stability. Through Sentaurus TCAD simulation, it is found that the charge transport layer selectively blocks carrier transmission, thereby reducing dark current. The simulation results are in good agreement with experimental data, providing theoretical guidance for a more in-depth understanding of the intrinsic physical mechanisms.
GEOPHYSICS, ASTRONOMY, AND ASTROPHYSICS
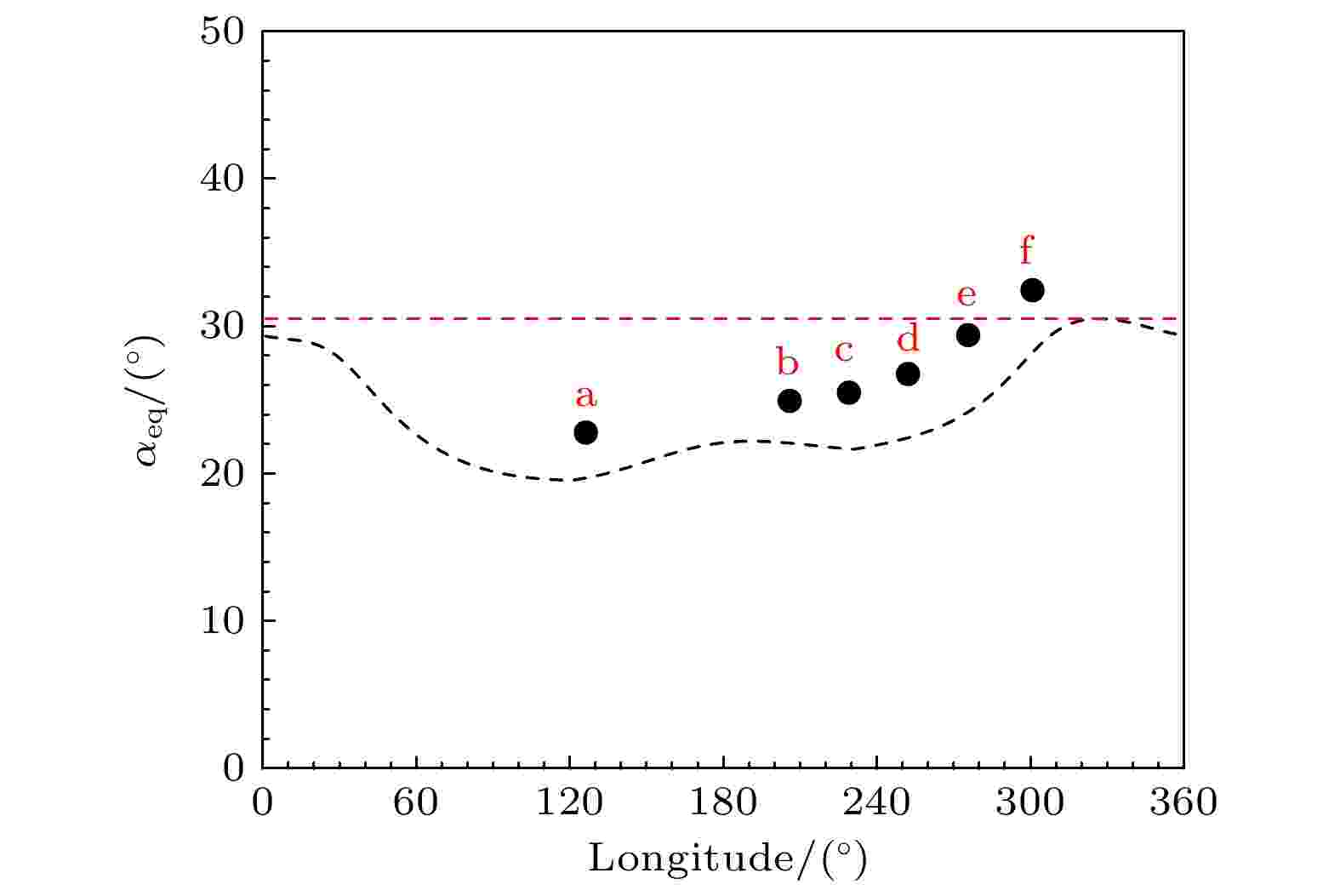
EDITOR'S SUGGESTION
2024, 73 (20): 209401.
doi:10.7498/aps.73.20240975
Abstract +
Very low frequency (VLF) signals emitted by worldwide spread ground-based man-made transmitters mainly propagate in Earth-ionospheric waveguides and are used for submarine communication. A portion of these signals penetrate the ionosphere and leak into the magnetosphere when the ionospheric electron density decreases on the nightside due to the attenuated sunlight. The VLF transmitter signals in the magnetosphere can scatter electrons with energy of several hundred keV in the inner radiation belt into the drift loss cone through cyclotron resonance. This is an important loss mechanism for electrons in the inner radiation belt and plays an important role in transferring energy and mass from magnetosphere to ionosphere. Electrons scattered by transmitter signals exhibit a “wisp” characteristic inL-Ekspectrum, satisfying the first-order cyclotron resonance relationship between the electrons and the transmitter signals. The “wisp” spectrum can be clearly observed by low earth orbit satellites, presenting opportunities to study wave-particle interactions in near-Earth space. In this study, using the Drift-Diffusion-Source model, we reproduce the “wisp” spectrum formed by scattering effects of NWC transmitter signals observed by DEMETER satellite on March 19, 2009. Our simulation results suggest that the equatorial pitch angle of electrons, observed by DEMETER, varies with the longitude, resulting in distinctions in the observed “wisp” spectrum along different longitudes. Specifically, as the satellite approaches South Atlantic Anomaly (SAA) region, both the energy range and flux level of the observed “wisp” spectrum gradually increase. When the previously studied wave normal angle model (with a central wave normal angle of 60°) and the background electron density model are used, the energy range of the simulated “wisp” spectra is higher than the observed value. Adjusting the central wave normal angle to 40° or increasing the background density by a factor of 1.3, the simulated results accord well with the observations. Our results elucidate the scattering effect of NWC transmitter signals on electrons in the radiation belt, and emphasize the importance of analyzing the formation of “wisp” spectrum for understanding wave-particle interactions in near-earth space. Additionally, the Drift-Diffusion-Source model can be used to study wave-particle interactions in the inner radiation belt, helping to develop radiation belt remediation technology.